Successor of Today’s Mobile internet: The Metaverse
The Metaverse is the next paradigm shift after the mobile internet. The Metaverse will utilize head-mounted devices (HMDs) and extended reality (XR) as the medium to connect avatars and users in the real world. Furthermore, the Metaverse is supposed to provide gamified experiences around emerging Web 3.0 technologies and is anticipated to be the origin of the Multiverse.
The Metaverse will utilize the following hardware and software components: Hand and non-hand-based input devices, scene/object/sound/speech recognition and synthesis, motion rendering, and HMDs. Furthermore, gamification will encompass the activity and story around emerging Web 3.0 non-fungible tokens (NFTs).
Precursor of Tomorrow’s Multiverse
The Metaverse will be the precursor of the Multiverse. While the Metaverse primarily focuses on virtual reality (VR) and augmented reality (AR), the Multiverse offers eight different types of reality and advanced XR experience realms, including but not limited to VR and AR, as illustrated in Fig. 1.
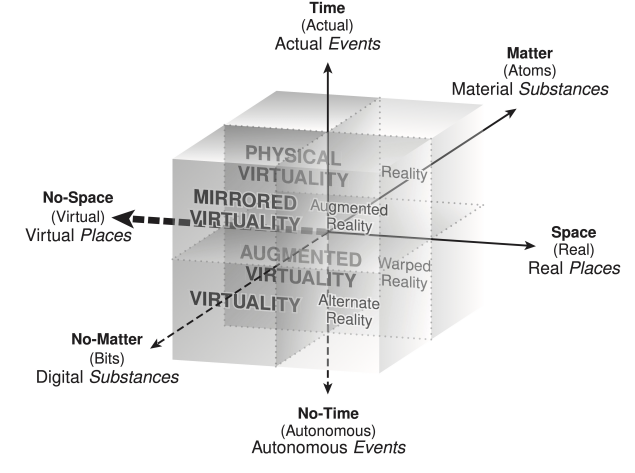
Fig. 1. The Multiverse as an architecture of advanced XR experiences [1].
The Multiverse consists of three pairs of variables, each with two opposite physical/digital dimensions – Space/No-Space, Time/No-Time, and Matter/No-Matter – which give rise to a total of eight realms, each offering a different type of reality. Each combination of the six variables yields a distinct realm: 1) Reality: The realm Reality consists of the variables Time/Space/Matter. We experience this realm as real life. 2) Virtuality: The exact opposite of reality consists of the variables No-Time/No-Space/No-Matter. 3) Augmented Reality: It is characterized by the variables Time/Space/No-Matter. 4) Augmented Virtuality: This realm is characterized by the variables No-Time/No-Space/Matter, which is widely used for realizing digital twins by taking something material and tactile and using it to augment an otherwise virtual offering. 5) Alternate Reality: Alternate Reality comprises the variables No-Time/Space/No-Matter. Its essence lies in constructing a digital experience and superimposing it onto a real place to create an alternate view of the physical reality. 6) Physical Virtuality: It takes real-world objects and designs them virtually. The resultant Time/No-Space/Matter experience occurs when virtually designed artifacts take material shape. 7) Warped Reality: This realm consists of the variables No-Time/Space/Matter. It takes an experience firmly grounded in Reality and shifts only one variable, moving the event from actual to autonomous time. It requires the offering to play with or manipulate time in some way that makes it clearly distinct and different from normal experience. The involved reality-based time travel happens whenever experiences simulate another time in the past or future. 8) Mirrored Virtuality: It is characterized by the variables Time/No-Space/No-Matter. Inside Virtuality, operating via some sort of avatar, a user generally remains free to do whatever she wishes to do, whereas inside Mirrored Virtuality she remains tied to what is happening in the real world, in real time, moment by moment.
6G vs. 5G
5G wireless communication networks have already been deployed with the aim of reaching high data rates. With rapid increases in the number of smart devices and emergence of new applications, more reliable communications with lower latency is required. Since 5G networks are unlikely to sustain the data traffic explosion, researchers shifted their focus on the next generation of 6G mobile networks. Most of the KPIs that are applied for evaluating 5G will be still valid for 6G, though scaled up by a factor of 10, 100, or even 1000, as illustrated in Fig. 2.
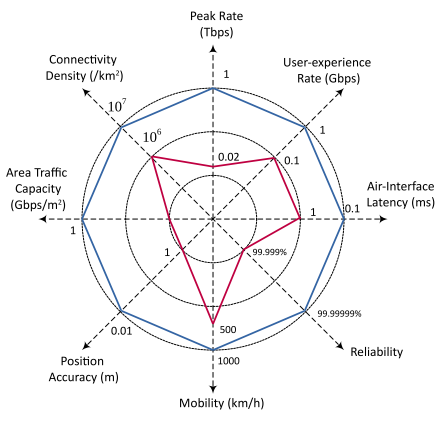
Fig. 2. Quantitative comparison of KPI requirements between 6G and 5G [2].
6G Mobile Networks
Four-Tier Network Architecture: Space-Air-Ground-Underwater
By integrating underwater networks, 6G is expected to convert current 3-tier space-air-ground networks into 4-tier networks that will have near instant and unlimited super-connectivity in the sky, at sea, and on land.
- Space-Network Tier:
This network tier will support space Internet services in space travels and provide wireless coverage via satellites. For long-distance intersatellite transmission in free space, laser communications represent a promising solution. The integration of terrestrial and non-terrestrial networks has a number of challenges: a) large propagation delays, b) Doppler effect due to fast moving satellites, and c) severe path loss for mmWave transmissions.
- Air-Network Tier:
This tier works in low-frequency, microwave and mmWave bands to provide more flexible and reliable connectivity for urgent events or in remote areas by densely employing flying base stations, such as unmanned aerial vehicles (UAVs).
- Terrestrial-Network Tier:
Similar to 5G, this network tier will still represent the main solution for providing wireless coverage for most human activities. It will support low-frequency, microwave, mmWave, and Terahertz (THz) bands.
- Underwater-Network Tier:
This tier aims at providing coverage and Internet services in support of broad-sea and deep-sea activities for military or commercial applications. Given that water exhibits different propagation characteristics, acoustic and laser communications can be used to achieve high speed data transmission for bidirectional underwater.
Standardization Roadmap
In July 2018, a focus group “Technologies for Network 2030” was established under ITU-T. This group intends to study and review existing technologies, platforms, and standards for identifying the gaps and challenges towards the capabilities of networks for the year 2030 and beyond, with the emergence of novel technologies such as holographic applications, Tactile Internet, multi-sense networks, and digital twin. According to the empirical timeline, the ITU-R section will initiate the study of 6G vision and will publish the requirements for IMT for 2030 in the middle of the 2020s and step into the evaluation phase afterwards, as illustrated in Fig. 3.
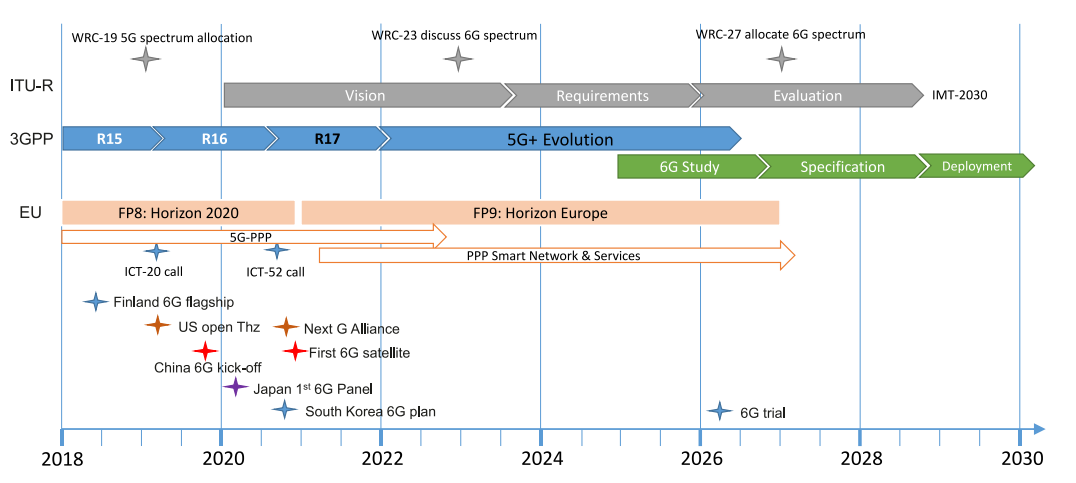
Fig 3. Predicted roadmap of research, definition, specification, spectrum regulation, development, and deployment for 6G mobile systems [2].
SDO Efforts
A number of standard developing organizations (SDOs) have started their efforts to develop standards for future 6G mobile networks. Among others:
- European Telecommunication Standard Institute (ETSI)
- Next Generation Mobile Networks (NGMN) Alliance
- Alliance for Telecommunications Industry Solutions (ATIS)
- Next G Alliance
- Association of Radio Industries and Businesses (ARIB)
- 3rd Generation Partnership Project (3GPP)
- IEEE Future Networks (FN) Initiative
- ITU-T Focus Group on Technologies for Network 2030 (FG-Net-2030)
Next G Research
One of the most interesting SDOs is the Next G Alliance. Note that Next G research includes but is not limited cellular and wireless technologies and goes beyond 6G standardization efforts. Next G research is a disruptor, because it involves players and stakeholders, most notably many of over-the-top (OTT) Internet players that have already embraced the Metaverse.
Next G Alliance Working Groups
The Next G Alliance has the following 6 working groups: 1) National 6G roadmap, 2) technology, 3) green G, 4) societal and economics needs, 5) spectrum, and 6) applications. The national 6G roadmap group integrates the results from other working groups and interprets them. This group is also responsible for defining the timeline. The Next G Alliance also has a steering group and a full members group. The full members group is responsible for strategy, operating procedures, and membership. The steering group defines the working groups and implements the strategic direction of the full members group. Further, there is a technical program office that synchronizes the actions of all groups. The Next G Alliance’s objectives are as follows: 1) Market readiness, 2) raise government research funding, and 3) progress North America’s ecosystem for developing Next G [3].
Next G Alliance Goals
The Next G Alliance aims at realizing the North American 6G vision by pursuing the following six goals: 1) Trust, security, and resilience: Next G networks should be advanced, fully trusted by everyone, and available under all conditions. It includes: a) service availability, b) security and privacy, c) data protection, and d) resilience in networks and services; 2) enhanced digital world experience (DWE): It consists of multi-sensory experiences that builds human-to-machine or machine-to-machine interactions. It tries to improve work, education, and entertainment. Moreover, it tries to improve everyday life, quality of experience, as well as the quality of critical services, e.g., humanized robotic care. One of the special features of DWE is that it enables users to be anywhere at any time via mixed reality (MR). Extended reality (XR) is enabled by means of remote sensing, haptic feedback, and actuation, and plays a crucial factor in realizing DWE. DWE removes the flat-screen approach and instead uses multi-dimensional, multi-party, and multi-sensory technologies [3].
Novel Services and Disruptive Applications
Enhanced Services
As shown in Fig. 4, three new services are considered to meet the KPI requirements of different 6G use cases: 1) Ubiquitous MBB (uMBB): It supports global ubiquitous connectivity. This new service will be the foundation of digital twin, pervasive intelligence, enhanced on-board communications, and global ubiquitous connectivity. 2) Massive URLLC (mULC): mULC combines the characteristics of both mMTC and URLLC, which will facilitate the deployment of massive sensors and actuators in vertical industries. 3) Ultra-reliable low latency broadband communication (ULBC): ULBC supports applications like HTC-based immersive gaming, extended reality (ER), Tactile Internet, multi-sense experience, and pervasive intelligence [2].
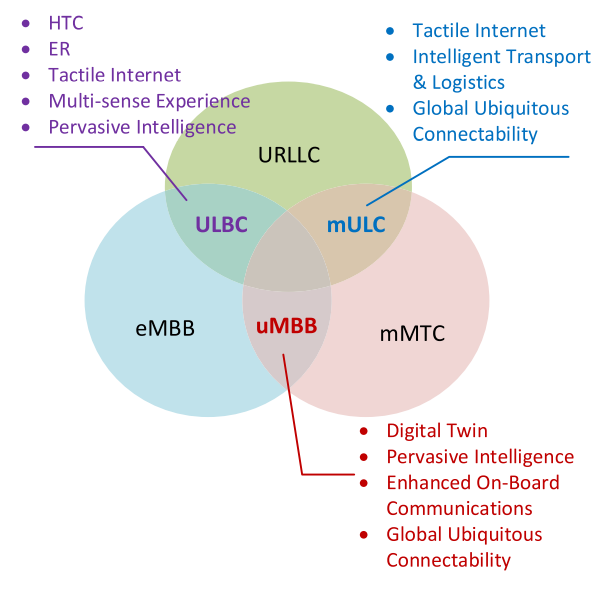
Fig. 4. In addition to 5G services (eMBB, ULRRC, and mMTC), three enhanced services named uMBB, ULBC, and mULC are considered in 6G [2].
Disruptive Applications
XR includes a series of immersive technologies, including VR, AR, and MR. XR will be the next-generation mobile computing platform that realizes the entire reality-virtuality continuum for the extension of human experiences. As shown in Fig. 5, user experiences expand from one single sense in text communication to 3 senses in VR/AR and soon to 5 senses in hologram communications.
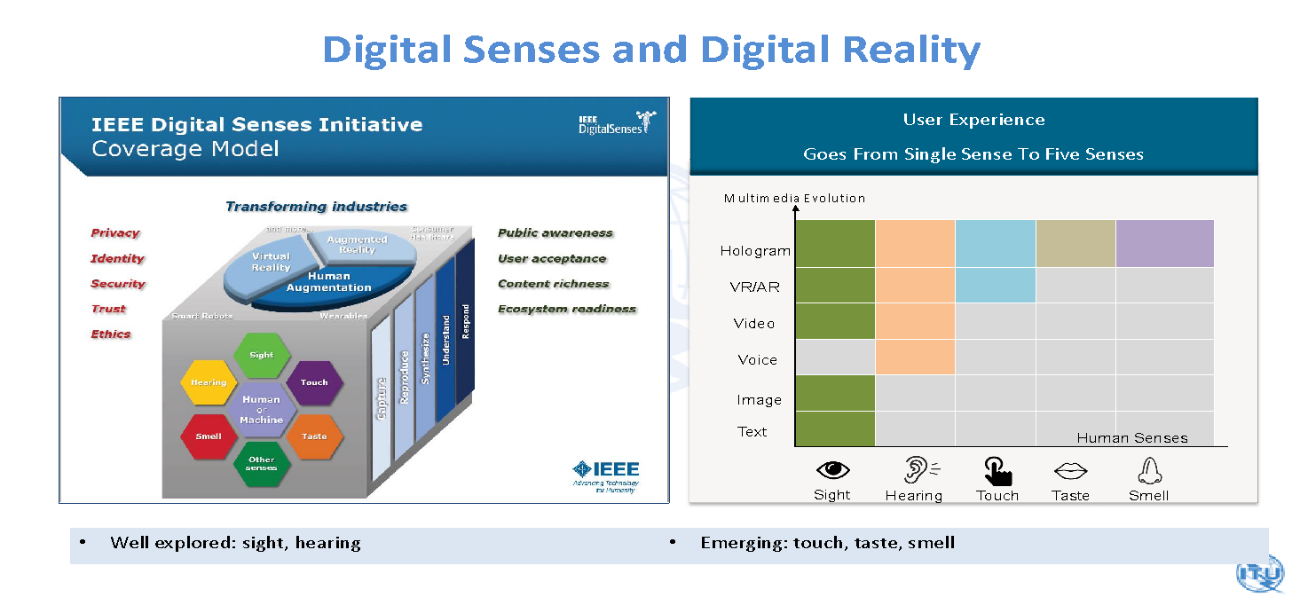
Fig. 5. Digital senses and digital reality [Source: ITU-T].
Multi-Sense Experience
Humans hav five senses (sight, hearing, touch, smell, and taste) to interact with the external environment. However, current communications focus only on visual (text, image, and video) and acoustic (audio, voice, and music) media. The involvement of the senses of taste and smell can create fully immersive experiences, which may bring forth new services, e.g., in food and texture industries. Furthermore, the application of haptic communications will play an important role and give rise to a wide range of novel applications such as remote surgery, remote controlling, and immersive gaming.
Digital Twin
Digital twin is used to create a comprehensive and detailed virtual copy of a physical object. The softwarized copy is equipped with a wide range of characteristics, information, and properties related to the original object. Such a cybertwin is then used to manufacture multiple copies of an object with full automation and intelligence.
Digital twins can be categorized into monitoring digital twins, simulation digital twins, and operational digital twins. A monitoring twin supports monitoring the status of a physical system (e.g., autonomous car dashboard), whereas a simulation twin uses various simulation tools and machine learning schemes to provide insights about future states. Conversely, an operational twin enables system operators to interact with a cyber-physical system and perform different actions in addition to analysis and system design. To design 6G systems, an operational digital twin allows users to enable efficient interactions among various players of 6G. This is not possible without an operational twin, because other twins (e.g., simulation and monitoring twins) enable users to only monitor or analyze the cyber physical system without controlling the system in real time [6].
A digital twin is a virtual representation of the elements and dynamics of a physical system. Digital twins for 6G can be used to emulate an entity/function (e.g., IoE device or edge server) or an IoE service (i.e., XR-based healthcare). To design 6G systems, digital twins allow users to enable efficient interaction among various players of 6G [9].
Pervasive Intelligence
Intelligent tasks rely on traditional computation-intensive AI technologies such as computer vision, simultaneous localization and mapping (SLAM), face and speech recognition, natural language processing, motion control, to name a few. To overcome their stringent computation, storage, power, and privacy constraints on mobile devices, 6G networks will offer pervasive intelligence in an AI-native manner by utilizing distributed computing resources across the cloud, mobile edge, end-devices, and cultivating communication-efficient machine learning training and interference mechanisms. In addition to computation-intensive tasks, pervasive intelligence also facilitates time-sensitive AI tasks to avoid the latency constraint of cloud computing when fast decisions or responses to conditions are required [2].
Key Enabling Technologies
Terahertz Communications
Terahertz communications is one of the key enabling technologies of 6G that can support peak data rates of 1 Tbps, peak spectral efficiency of 60 bps/Hz, latency of 0.1 ms, and reliability of 10^(-9) packet error rates. Terahertz communication can be used in different use cases such as Tbps WLAN system (Tera-WiFi), Tbps Internet-of-Things (Tera-IoT) in wireless data centers, Tbps integrated access backhaul (Tera-IAB) wireless networks, ultra-broadband THz space communications (TeraSpaceCom), wireless networks-on-chip communications (WiNoC), and wireless connections in networks of nanomachines such as in an Internet of NanoThings (IoNT). Furthermore, with the help of AI one can build THz holographic, haptic, and telepresence communications, which provide a significant progress in entertainment and education [7].
Blockchain
Blockchain is viewed as another 6G key technology. Blockchain is a distributed ledger in which information is stored as a chain of data blocks. It provides both easy deployment and high security of the network, including data integrity, non-repudiation, and auditability. Consensus in blockchain makes sure that all nodes agree on the network state. A blockchain can be either public, a consortium, or private. In public blockchains, any node can join, leave, read, and write. In a consortium blockchain, the ability to write is exclusive to a group of organizations. Conversely, in a private blockchain, the write access right is owned by a single organization. Proof-of-work (PoW) algorithms provide a great amount of security, though when the nodes are resource-constrained they may not perform the same. By increasing the number of verifiers via delegated proof-of-stake (DPoS), communications can be rendered more secure. In DPoS, the communication overhead and the time needed to reach a consensus is higher than in PoW.
Further, blockchain can be utilized to achieve better spectrum management, infrastructure and asset management, computing power, data storage management, AI model parameter management, data integrity, non-repudiation, and auditability. For example, in spectrum management, smart contracts may be used in a situation where licensed spectrum owners and unlicensed spectrum operators are able to cooperate [8].
Holographic Type Communication (HTC)
Holograms provide visual cues by observing 3D objects with the naked eye. Remote rendering high-definition holograms through a mobile network are able to create truly immersive experiences. For example, holographic telepresence allows remote participants to be projected as holograms into a meeting room and allow attendees of online training or education to interact with ultra-realistic objects. At the downside, HTC has huge bandwidth demands on the order of Tbps, even with image compression. HTC also requires ultra-low latency for true immersiveness and high-precision synchronization for reconstructing holograms.
Tokenomics
The tokenization process can be described as the encapsulation of value in tradeable units of account, called tokens or coins. In other words, tokenization represents a form of digitalization of value and, just like the Internet enabled the free and fast circulation of digitized information, so does blockchain allow the free and borderless flow of digitized value. Once tokenized, every kind of value can be managed as a digital asset, whose unit of account is a dedicated virtual token. Such virtual tokens can be minted by any individual or an organization that defines the set of rules governing them, e.g., token features, monetary policy, and user incentives.
The tokenization process can be further described as the creation of a self-governed tokeconomic system, whose rules are programmed by the token issuer. In 6G, we have a shift from economics to tokenomics. In economics, innovation proceeds and propagates by introducing a change in the context of set rules and by observing how such a relatively rigid framework reacts to the change. Therefore, the outcome of the introduced innovation is assessed, at first, on a predictive basis. Conversely, in tokenomics, innovation is put forward by designing the rules governing the playground in a way that the stakeholders’ behavior aligns with the goal pursued [10].
Open Research Challenges
Although 6G is the focus of many researchers in the world, there are still open challenges that need to be studied. These open challenges include:
- THz Communications
Efficient THz Channel Measurement Systems: Most of the existing measurement methods are adapted from systems designed for low-frequency bands, i.e., mmWave. However, the special properties of THz waves motivate more advanced and flexible systems to handle significant path loss, ultra-large bandwidth at high frequency, as well as short coherence time. Based on such systems, extensive channel measurement studies should be conducted for various scenarios, including but not limited to unmanned aerial vehicles assisted wireless communications as well as VR/AR/MR/XR.
- AI and Machine Learning (ML)
Data Availability and Benchmarking: One of the challenges in wireless networks is data availability. Data availability is related to the problem of identifying a common and accepted set of data (e.g., channel realizations) with the goal of testing and benchmarking ML algorithms. Normally, algorithms and proposals are tested using approved underlying physical models (e.g., urban macro-/microcell channel models), evaluation methodologies, and calibrated simulators. Cellular networks have no standard data set to train and benchmark an ML algorithm. Therefore, a synthetic or software-generated data set is of great importance to train and benchmark ML algorithms and to agree on a common evaluation methodology to rank proposition and standard algorithms. Identifying a set of critical KPIs in wireless networks is another crucial task for ML standardization. It is necessary to design a set of metrics to classify and rank ML algorithms and their performance. Conventional metrics such as throughput and signal-to-interference-plus-noise ratio might not be sufficient because small improvements of these values might come at the expense of increased complexity and energy consumption.
- Blockchain Implementation
Blockchain Techniques: They could be utilized for reducing convergence times in very large blockchain networks. Smart contract optimization techniques are necessary for decreasing block size and consensus latency. Smart contracts should also be written extremely carefully to make them less vulnerable to hackers. Larger network sizes translate into larger storage requirements. Off-chain storage may be used along with a signature associated with the block that can be stored on the chain. Less resource-intensive consensus algorithms: They offer promising solutions without compromising security features. There is a clear need for less resource-intensive consensus algorithms.
- Holographic-Type Communications
User Interactivity: Even with compression, holograms require massive bandwidth. To cope with this, the current tendency is to come up with clever techniques to reduce data that need to be transmitted by eliminating parts of the content that go unnoticed by a user. For example, some areas may be obstructed from the user’s viewpoint or some angles may not come into view based on the user’s position. Schemes that take advantage of these aspects can dynamically decide which parts of the content to stream and at what quality at any given time. However, the effectiveness depends on the ability to predict the user’s movement in order to rapidly adapt the data as needed. This is referred to as the user interactivity challenge, which enforces not only ultra-high bandwidth but also ultra-low latency to ensure interactivity with the content.
Ultra-Low Delay: Achieving ultra-low latencies over long distances is nearly impossible due to physical limitations imposed by the speed of light. Ultra-low latency is crucial for true immersiveness to alleviate simulator sickness, especially if HMDs are involved. Furthermore, applications involving real-time communications impose stringent latency requirements on the transmitted stream [12].
- Tokenomics
Generalizing Token-Represented Value: The value represented by a token observable out in the wild is diversified and cannot be described univocally: it can be either the right to have a discount on an exchange rate, the proof of ownership, the reward for solving a mathematical problem to validate the next block of the chain, among many other examples. The challenge is to generalize the definition of token-represented value by defining a common trait that all these expressions of value share. Looking deeply into the source of token-encapsulated value, the concept of trust occurs over time and again.
Conflict between Different Token Features: If an investor as a token holder wants to wait until the value goes up, she will probably not be able to use it. On the other hand, the more tokens are used, the higher their value is. The challenge is to find a way to solve this potential conflict within the design of the token economy.
Researchers
Graduate Student
Advisor
Publications
- M. Maier, N. Hosseini, and M. Soltanshahi,
“INTERBEING: On the Symbiosis between INTERnet and Human BEING,”
IEEE Consumer Electronics Magazine, Special Issue Metaverse and eXtended uniVerse (XV): Opportunities and Challenges for Consumer Technologies, to appear
- M. Maier, M. Soltanshahi, and N. Hosseini,
“Metaverse as the New Eleusis 2.0: Are We in the Midst of the Next Renaissance?,”
IFSA Publishing, Blockhain and Cryptocurrency, vol. 1, no. 1, pp. 1-17, Sept. 2023
References
[1]
|
A. Ebrahimzadeh and M. Maier, “Toward 6G: A New Era of Convergence”, Wiley-IEEE Press, 2021.
|
[2]
|
W. Jiang, B. Han, M. A. Habibi, and H. D. Schotten, "The Road Towards 6G: A Comprehensive Survey," IEEE Open Journal of the Communications Society, vol. 2, pp. 334-366, 2021.
|
[3]
|
“Roadmap to 6G,” 2022. [Online]. Available:" https://nextgalliance.org/.
|
[4]
|
G. Fettweis and H. Boche, “6G: The Personal Tactile Internet—And Open Questions for Information Theory,” IEEE BITS the Information Theory Magazine, vol. 1, no. 1, pp. 71–82, 2021.
|
[5]
|
S. Bera, H. Das, S. Nayak, and R. Patgiri, “Future Tactile Internet: Issues, Challenges and Applications,” in Proc., IEEE International Conference on Signal Processing,Computing and Control, pp. 625–630, 2021.
|
[6]
|
L. U. Khan, W. Saad, D. Niyato, Zh. Han, and Ch. S. Hong, “Digital-Twin-Enabled 6G: Vision, Architectural Trends, and Future Directions”, Nov. 2021. [Online]. Available : arXiv:2102.12169.
|
[7]
|
I. F. Akyildiz, C. Han, Z. Hu, S. Nie, and J. M. Jornet, “Terahertz Band Communication: An Old Problem Revisited and Research Directions for the Next Decade (Invited Paper),” IEEE Transactions on Communications, 2022.
|
[8]
|
A. H. Khan et al., “Blockchain and 6G: The Future of Secure and Ubiquitous Communication,” IEEE Wireless Communications, vol. 29, no. 1, pp. 194–201, 2022.
|
[9]
|
L. U. Khan, W. Saad, D. Niyato, Z. Han, and C. S. Hong, "Digital-Twin-Enabled 6G: Vision, Architectural Trends, and Future Directions," IEEE Communications Magazine, vol. 60, no. 1, pp. 74-80, 2022.
|
[10]
|
P. Freni, E. Ferro, and R. Moncada, "Tokenization and Blockchain Tokens Classification: a morphological framework," in Proc., IEEE Symposium on Computers and Communications (ISCC), pp. 1-6, 2022.
|
[11]
|
A. Beniiche, S. Rostami, and M. Maier, "Robonomics in the 6G Era: Playing the Trust Game With On-Chaining Oracles and Persuasive Robots," IEEE Access, vol. 9, pp. 46949-46959, 2021.
|
[12]
|
A. Clemm, M. T. Vega, H. K. Ravuri, T. Wauters, and F. D. Turck, "Toward Truly Immersive Holographic-Type Communication: Challenges and Solutions," IEEE Communications Magazine, vol. 58, no. 1, pp. 93-99, 2020.
|
|