|
Optical Coding (OC) enabled Carrier-Grade Ethernet Networks
Motivation
Low-cost and reliable optical metropolitan and access networks are poised to be the next major development in communications. Intense research is currently focused on bridging the gap between the large backbone bandwidth resources and the high client processing speeds. The ability of recently proposed architectures based on the combination of WDM EPONs with Ethernet-based metro core architectures to incorporate gradual low-cost WDM-technology upgrades makes them leading contenders for a future all-optically integrated metro-access network. Nonetheless, such proposals share one major shortcoming with most proposed metropolitan and access architectures: They offer no framework for Operation, Administration, and Maintenance (OAM) procedures, which are key features of carrier-grade networks.
Optical Coding (OC) holds the potential to remove that limitation. Despite more than two decades of research, the feasibility of Optical Code Division Multiple Access (OCDMA) remains in question. As a fresh approach on encoding technology, OC promises to reverse long-held OCDMA paradigms. In OC, the encoded pulses may carry a range of control- and management-plane related information such as the identities of label-switched paths, multicast groups, or optical tree branches. Besides, the simpler OC configurations combine more easily with conventional TDM and WDM systems and represent a smoother upgrade path towards all-optical networks. Yet, the scarce OC proposals have so-far included little network-layer evaluation. Moreover, no contribution proposes the systematic use of OC as an OAM solution.
OC Principles
Optical Code Division Multiplexing (OCDM) is a multiplexing procedure by which each communication channel is distinguished by a specific optical code rather than a wavelength or time-slot. An encoding operation optically transforms each data bit before transmission. At the receiver, the reverse decoding operation is required to recover the original data. The encoding and decoding operations alone constitute
Optical Coding.
Encoding involves multiplying the data bit by a code-sequence either in the time-domain, the wavelength-domain, or a combination of both. The latter method is called two-dimensional coding (2D-coding). Fig. 1 illustrates the three coding principles. In a time-encoded signal, the bit is split to smaller time-components called chips. Time-domain coding that manipulates the phase of the optical signal is usually bipolar and requires phase-accurate coherent sources. Alternatively, positive encoding manipulates the power of the optical signal but not its phase and typically uses incoherent sources. In wavelength-domain coding, transmitted bits consist of a unique subset of wavelengths forming the code. 2D-coding combines both wavelength selection and time spreading. A data bit is encoded as consecutive chips of different wavelengths, the unique wavelength sequence constituting the code. Decoding consists of applying the reverse time/wavelength operations. Regardless of the coding domain, the coding operation broadens the spectrum of the data signal, hence the designation of
spread spectrum.
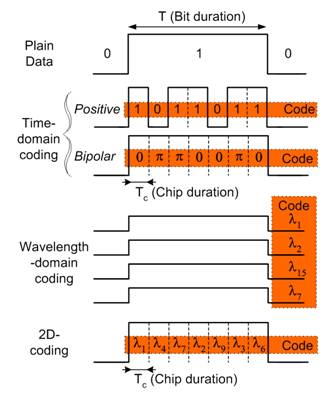 Fig. 1. Optical Coding Dimensions.
A vast array of physical-layer methods have been proposed to implement Optical Coding. This gives rise to alternative classifications using component characteristics rather than the coding dimension. For instance, employing incoherent vs. coherent light sources is often used to classify OCDMA systems as that choice has important cost and performance implications. Note that the choice of coherent sources does not always imply that chips are phase- rather than power-modulated. In addition, whether the encoding occurs in fiber, a planar lightwave circuit (PLC), or an out-of-fiber external device has an important impact on system design.
OC and OCDMA
Optical Code Division Multiple Access (OCDMA) is the use of OCDM technology to arbitrate channel access among multiple network nodes in a distributed fashion. OCDMA is based on the principle that codes are mapped to the identities or addresses of users following a
code-user relation. Accordingly, OCDMA was initially proposed to implement ultrafast asynchronous broadcast LANs. More recently, OCDMA has been viewed as a candidate technology for future PON access networks. OCDMA has considerable potential on the networking level. A well designed OCDMA access network eliminates channel contention. In other words, provided interference is controlled, any upstream or downstream connection may be established asynchronously with no collisions or blocking, entailing substantial networking implications.
Nevertheless, OCDMA deployment faces considerable physical-layer barriers. OCDMA implementations span a large array of disparate optical and optoelectronic component technologies that are mostly experimental. Indeed, attempts to control interference and noise at high data bitrates generally yield extremely complex and costly encoder architectures that are not suitable for deployment. In addition, many system design issues such as power budgeting and multirate operation are yet unverified experimentally. Furthermore, OCDMA propositions suffer from a lack of transitional models that take into account legacy systems: Entire OCDMA networking systems are usually presented as bulk replacement alternatives.
Optical Coding (OC) represents a more practical approach to encoding in the optical domain. In OC, codes may correspond to network-layer information other than user identity. In a typical example of this
code-information relation, codes denote optical paths in MultiProtocol Label Switching (MPLS). Codes can also represent optical control and signaling messages in Optical Burst Switching (OBS), network fault detection, and maintenance. In such applications, Optical Coding achieves a form of optical information processing. It brings network-layer operations to the optical medium, further reducing the burden of electronic processing. OC processing rates are limited only by the speed of light in the encoding/decoding device.
OC Label Switching is among the most promising implementations of Optical Coding. In an OC label switched network, optically-encoded pulses are added to fixed-length packets as headers that specify the route or label-switched path (LSP). In an OC Label Switch (OCLS), each output port is controlled by a header-sensitive switching device called an Optical Code Gate (OC-gate). OC-gates allow only packets with a specific header (OC-label) through. Fig. 2 (a) depicts a simplified OC-gate architecture. The OC-label is tapped at the OC-gate input and fed to the OC-gate decoder while the packet enters a delay line. If the OC-label matches the decoder, the resulting optical pulse triggers an ON-OFF electro-optical switch in time to let the packet through. Only packets with an OC-label correlating with the decoder can cross the OC-gate. The switch maintains the optical path open for the duration of one packet.
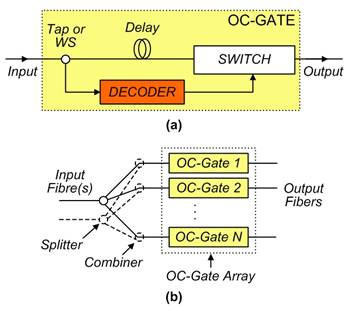 Fig. 2. OC Label Switch Architecture.
In an OCLS, packet power is split among router outputs, as shown in Fig. 2 (b). Only the output with the same code as the OC-label forwards the packet, thus completing the switching process. In a more integrated approach, the OC-label may be tapped at the label switch input and fed to an array of decoders, each of which controls a specific output. Although this architecture uses one common delay line for all outputs, we preferred to introduce individual OC-gates due to their expected versatility, particularly for optical network control and signaling. The OC-gate array in Fig. 2 (b) may be duplicated for each input fiber on a separate switching plane or reused by more than one input fiber (dashed connections). Amplification and pulse reshaping stages may also be required. OC-labels may be in-band or out-of-band with the optical packet. Out-of-band labels are simpler to implement since they can be easily stripped using Wavelength/Waveband Selective (WS) devices or be transmitted alongside the packet rather than prior to it.
Research Direction
The envisioned research studies aim at establishing OC as the next step in optical metro-access technology by developing an OC-upgraded Ethernet-based network architecture featuring novel OC-enabled control-plane operations and OAM processes.
The design of OC-enabled network and node architectures comprises the following aspects:
- Thorough study of OC and OCDMA to identify the major OC network-level strengths
- Modification of the considered architecture through integrating selected OC capabilities within a subset of transmitter, receiver, and routing modules.
- Verification of the impact of proposed OC upgrades through discrete-event simulation and experimentation.
The introduction of OC-enabled network control and management processes implies the following procedures:
- Comprehensive survey of state-of-the-art OAM procedures and efficiency metrics from scientific publications, standards, and industry R&D proposals.
- Study of the recently standardized carrier-grade Ethernet OAM procedures and the proposal of a set of complimentary OC-enabled OAM operations.
- Evaluation of the efficiency of performance-enhanced node and network architectures through analysis and simulation.
- Proof-of-concept experimentations using the laboratory’s state of the art Carrier-Grade Ethernet equipment.
This project is unique in defining the field of OC and investigating the full potential of OC applications for the control and management of all-optical access-metro networks. It is expected to enable optical network enhancements of significant scale with important ramifications on backbone networks and photonic processing applications. Furthermore, it reconciles a partly sceptical networking community with optical encoding and decoding technologies.
Researchers
Advisor
Collaborators
- Prof. Hussein T. Mouftah
- Prof. Lawrence R. Chen
Visitors
- Prof. Michael Scheutzow
- Tomaz Berisa
Graduate Students
Publications
- K. Fouli, J. Casse, I. Sergeev, M. Medard, and M. Maier, “Broadcasting XORs: On the Application of Network Coding in Access Point-to-Multipoint Networks”, Proc., International Workshop on Multiple Access Communications (MACOM), Dublin, Ireland, Nov. 2012
- T. Berisa, K. Fouli, M. Maier, and A. Bazant, “Adaptive Grant Extension in OC-Enhanced PONs”, IEEE Communications Letters, to appear
- K. Fouli, L. R. Chen, and M. Maier, “Time-, Wavelength-, and Code-Domain Optical Reflection Monitoring for Next-Generation Access-Metro Networks”, Computer Communications, to appear
- X. Liu, K. Fouli, R. Kang, and M. Maier, “Network-Coding-Based Energy Management for Next-Generation Passive Optical Networks”, IEEE/OSA Journal of Lightwave Technology, vol. 30, no. 6, pp. 864-875, March 2012
- M. M. Rad, K. Fouli, H. Fathallah, L. Rusch, and M. Maier, “Passive Optical Network (PON) Monitoring: Challenges and Requirements”, IEEE Communications Magazine, Special Issue on Advances in Passive Optical Networks, vol. 49, no. 2, pp. S45-S52, Feb. 2011
- K. Fouli, T. Berisa, and M. Maier, “Optical Coding for Enhanced Real-Time Dynamic Bandwidth Allocation in Passive Optical Networks”, IEEE/OSA Journal of Lightwave Technology, vol. 27, no. 23, pp. 5376-5384, Dec. 2009
- K. Fouli, L. R. Chen, and M. Maier, “Optical Reflection Monitoring for Next-Generation Long-Reach Passive Optical Networks”, Proc., IEEE Photonics Society Annual Meeting, Belek-Antalya, Turkey, Oct. 2009
- K. Fouli, M. Maier, and H. T. Mouftah, “Optical Code Reflection Monitoring for Improved Resilience in Next-Generation Carrier-Grade Ethernet Access-Metro Networks”, Proc., SPIE/IEEE Photonics North, Quebec, QC, CANADA, May 2009
- K. Fouli and M. Maier, “The Road to Carrier-Grade Ethernet”, IEEE Communications Magazine, OFC/NFOEC Special Issue, vol. 47, no. 3, pp. S30-S38, March 2009
- K. Fouli, S. Aissa, and M. Maier. “Native Multicasting in a 2D-OCDMA PON”, IEEE Comm. Letters, vol. 12, no. 9, pp. 696-698, Sept. 2008
- K. Fouli and M. Maier, “OCDMA and Optical Coding: Principles, Applications, and Challenges”, IEEE Comm. Mag., vol. 45, no. 8, pp. 27-34, Aug. 2007.
|
|
|
| |